Novel Noise Monitoring Prototypes to Measure the Impact of Two-way Radio Earpiece Noise
Abstract
Current noise exposure measurement techniques fail to account for the noise emitted from a two-way radio earpiece and are impractical for highly variant and physically demanding work environments. Two novel prototypes were created to adapt a standard noise dosimeter for measuring noise levels when a two-way radio earpiece was used.
The first prototype was an attachment that fit over the microphone of a noise dosimeter and led up to the earpiece using acoustic tubing. The second prototype included a microphone in the earpiece. The two prototypes were laboratory tested using a mannequin system. When compared to the dosimeter equivalent, the corrected microphone prototype was more accurate than the corrected attachment prototype, with a mean difference of -0.2 dBA and -6.9 dBA, respectively. However, both were relatively consistent with the standard deviations of the difference between the corrected prototype and dosimeter equivalents being 0.76 dBA and 1.26 dBA, respectively.
The prototypes were used in a mechanized sorting facility and with police officers to measure the effect of using a two-way radio earpiece. In the sorting facility with ambient noise above 80 dBA, using an earpiece was protective, with measurements 3.6-7.9 dBA less than the levels measured when a two-way radio shoulder mounted speaker was worn instead. During the traffic stop scenario, the prototypes identified that wearing an earpiece appeared to be protective for the contact officer while it caused more noise for the cover officer, with mean noise levels of 8 or 12 dBA above the standard dosimeter.
Introduction
To facilitate communication and safety during occupational tasks, workers such as first responders, active-duty service members, construction workers, and industrial workers may utilize an earpiece attached to a two-way radio. First responders have an increased risk for noise-induced hearing loss (NIHL) (Lesage, Jovenin, Deschamps, & Vincent, 2009; Ide, 2011). However, their measured average exposures over a shift don’t always exceed the Occupational Safety and Health Administration (OSHA) action level (Tubbs, 1995; Gilbertson & Vosburgh, 2015). One explanation for the increased risk of NIHL may be the additional noise exposure that results from the use of a two-way radio with an earpiece.
The cause of the increased risk for NIHL among occupations using two-way radios with earpieces is difficult to discern. First, radio noise is unpredictable, transient, and varies acoustically from one instance to the next depending on communication needs and occupational tasks. Second, radios allow for adjustment of settings to accommodate background noise and listening preference, which varies across individuals (Keith, Michaud, & Chiu, 2008). Radio communication may need to be 2-15 dB above the ambient noise level for clear communication (Killion, 2002; Giguère, Behar, Dajani, Kelsall, & Keith, 2012). Individuals listening in noisy settings may increase the radio setting above the occupational damage risk criteria (Muchnik, Amir, Shabtai, & Kaplan-Neeman, 2012). Lastly, standard methods of noise dosimetry cannot measure the contributions of two-way radios.
A body of work exists evaluating noise exposure contributions from communication systems. Documented methods include the use of acoustical test fixtures, head and torso simulators, microphones in real ears, and indirect calculations (Dajani, Kunov, and Seshagiri, 1996; Giguère et al., 2012; Kunov, Giguere, &Simpson, 1989). A set of standards were developed to guide these methods of measurement (ISO, 2002; ISO, 2004; OSHA, 2013). Research teams utilized silicone tubing attached to a custom ear mold, measured voltage output of sound-generating devices, and utilized a small in-ear electronic microphone in the experimental setup in attempts to better measure noise from headsets and earpieces attached to sound-generating devices (Brammer, Yu, Bernstein, Peterson, Cherniack, & Tufts 2009; Muchnik et al., 2012; Shotland, 1996; Portnuff, Fligor, & Arehart 2013; Nélisse, Le Cocq, Boutin, Laville, & Voix 2015). However, these methods have not resulted in an affordable, portable, or practical option that can account for individual differences across employees as they actively navigate physically demanding work environments while using two-way radio earpieces for communication. This paper describes two novel prototype designs to support further development of affordable, portable noise monitoring devices for industry workers and first responders.
Research Methods
Prototype Designs
Two prototypes, attachment and microphone, were created as suitable options for measuring two-way radio exposure with an earpiece (U.S. Patent No. 10,560,776, 2020). The attachment prototype (Figure 1A) was designed to be simple with low costs associated with fabrication. The attachment prototype connected acoustic tubing from the dosimeter (Edge 5, 3M, St. Paul, MN, USA) microphone to the earpiece (Otto Engineering C101199-05 Quick Disconnect Acoustic Tube with Clear Eartip, OTTO Communications, Carpentersville, IL, USA). A plastic splitter in the shape of a “T” replaced the standard L-shaped connector that fit into a hole in the earpiece. The change in connector resulted in negligible changes in frequency response. The original acoustic tubing (C101199-5, OTTO Communications, Carpentersville, IL, USA) was connected to one arm of the T and then connected to the radio as normal. A second piece of acoustic tubing was attached to the other arm of the T and led to a hard plastic cover that slipped over the microphone of the noise dosimeter (Edge 5, 3M, St. Paul, MN, USA), after the windscreen was removed.
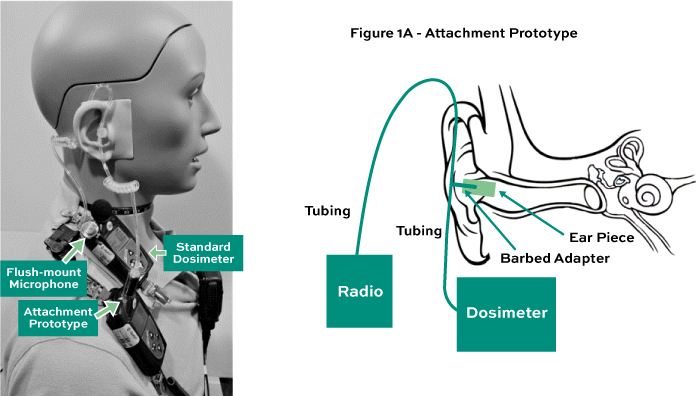
The microphone prototype design was more technologically advanced than the attachment prototype. For the microphone prototype (Figure 1B), the microphone on the noise dosimeter (Edge 5, 3M, St. Paul, MN, USA) was removed and a top-port micro-electro-mechanical systems (MEMS) (Invensense INMP510 Micro-Electrical-Mechanical System, dynamic range = 91 dB, frequency response= 0.06-20kHz) microphone was attached to the earpiece with silicone adhesive and a silicone retaining ring and wired to the noise dosimeter. The supply voltage and input signal to trigger calibration mode necessitated the design of a new circuit for the purpose of operating the MEMS microphone and amplifying its output to an appropriate level to interface with the existing circuit.
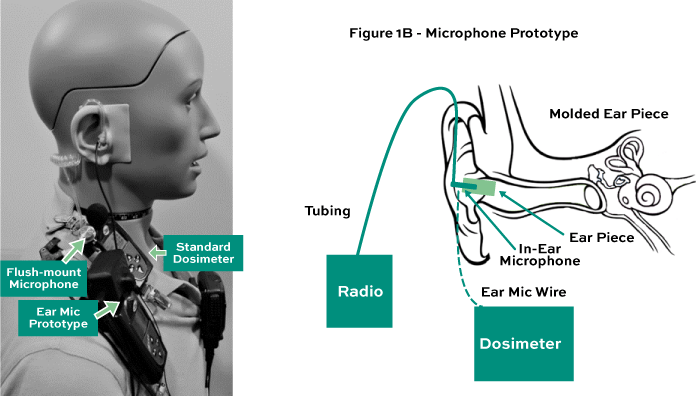
Due to design differences, the two prototypes did not respond the same to ambient noise and radio noise. When radio noise was transmitted through the tubing, it created resonant frequencies above 3,000 Hz. The resonance was similar to how acoustic tubing significantly influences mid-and high-frequency gain on hearing aids by the standing wave resonances within the tubing, causing additive or destructive interference at multiple frequencies (Taylor & Teter, 2009). Since the prototypes did not make significant changes to the mathematical calculations that were already programmed into the dosimeters, a two-step correction was needed to adjust the data the prototype collected only when noise was transmitted through the radio. Step one was to determine the mathematical equations to modify the one-minute measurements the prototype recorded. Because two-way radios are used intermittently throughout a work shift, the mathematical correction could not be applied across all one-minute measures. Step two identified which one-minute measurements required the mathematical correction when the two-way radio was in use.
Prototype Correction Step One
Laboratory Set-Up
Step one of the prototype correction occurred in a double-walled audiology booth with a mannequin system (KEMAR 45BC-2 Head & Torso with Mouth Simulator with Shore 00-55 Left and Right Ears, GRAS Sound & Vibration, Holte, Denmark). Data were digitized with a data acquisition system (SIRIUS MINI, Dewesoft, Trbovlje, Slovenia) connected to a laptop running data acquisition software (DewesoftX3, Dewesoft, Trbovlje, Slovenia). The internal mannequin microphone collected ⅓ octave band spectral data of the noise in the mannequin’s ear. The internal mannequin microphone results were converted into dosimeter equivalent noise levels using the ISO Standard 11904-2 (ISO 2004) for diffuse field so results could be compared to the prototype and standard dosimeter. For testing, three noise measurement devices (a prototype, a standard dosimeter, and a flush-mount microphone) were placed on the mannequin (Figure 1A and 1B). Either an attachment prototype or microphone prototype was placed on the mannequin. A standard dosimeter (Edge 5, 3M, St. Paul, MN, USA) was placed on the right shoulder of the mannequin next to the prototype dosimeter to measure the ambient noise in the room and represented the standard measure of noise exposure. A flush-mount microphone (47AD 1/2” CCP Flush-mount Microphone Set, GRAS Sound & Vibration, Holte, Denmark) was placed on the standard dosimeter, so the microphone was as close as possible to the standard dosimeter microphone and was connected to the data acquisition system. The flush-mounted microphone measured ⅓ octave band spectral data to serve as a quality control measure of ambient noise levels.
The standard dosimeter and the prototypes were programmed to have A-weighting, three-decibel exchange rate, 130 dB upper limit, and no threshold. All dosimeters were pre-calibrated and post-calibrated with a noise dosimeter calibrator (QC-10, 3M, St. Paul, MN, USA). A custom calibrator attachment was created for the microphone prototype so that it fit the appropriate location in the calibrator during calibration.
Two radio units (TK-5220, Kenwood Communications, Suwanee, GA, USA) were used during testing. One radio unit was clipped to the mannequin shirt waist and connected to the prototype. The earpiece was fit into the right ear of the mannequin for all tests. The partner radio remained outside the booth and served as the input for radio noise.
Pink noise served as the source of noise through the radio. To send pink noise through the radio, pink noise was emitted from an iPad (3rd generation iPad, Apple, Cupertino, CA) using the Audio Function Generator Pro application and transmitted to the two-way radio hand-held microphone outside of the sound booth. Every 30 seconds, the hand-held radio microphone key was released and then depressed again to avoid the warning beep that discharged from the hand-held radio microphone, if keyed for more than one minute.
Mathematical Equations
Tests were conducted to determine the relationship between the prototype measurements and the dosimeter equivalent values. The same test was conducted for both prototypes. Testing started with the radio connected to the prototype set so the prototype was measuring a level of at least 80 dBA. The radio setting was then increased by approximately three decibels for each subsequent measurement. Each decibel level was measured for three minutes. This continued until the radio setting was at 100%. Linear regression was then used to determine the relationship between the raw prototype decibel levels and the dosimeter equivalent levels (Figure 2). Equations 1 and 2 represent the attachment and microphone prototypes, respectively, where Lraw_attach is the raw prototype decibel level for the attachment prototype, and Lraw_mic is the raw prototype decibel level for the microphone prototype. Lcorr_attach is the corrected prototype decibel level for the attachment prototype, and Lcorr_mic is the corrected prototype decibel level for the microphone prototype.
Lcorr_attach=1.0448Lraw_attach-43.9 [1]
Lcorr_mic=1.0372Lraw_mic-27.6 [2]
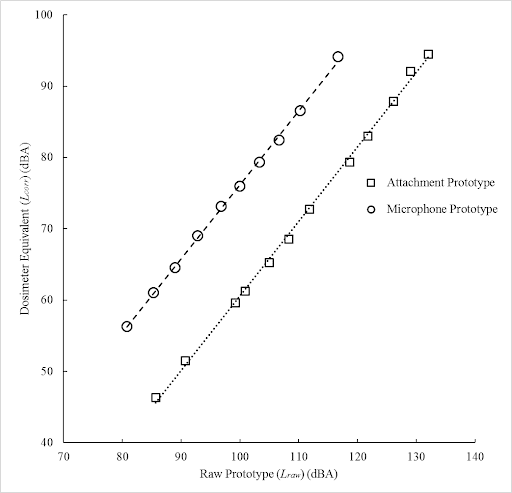
Correction Step One Laboratory Testing
Equations 1 and 2 were tested in the laboratory using the laboratory setup. Each prototype was tested at 50% and 100% radio setting, with and without ambient noise. Pink noise served as the source of noise through the radio and as the ambient noise source during laboratory testing. The ambient pink noise played in the sound booth 90 degrees azimuth to the mannequin over a portable speaker (Jam Plus Portable Speaker HX-P240GY, Frequency Response: 0.1-18 kHz, Jam Audio, Commerce Township, MI, USA) attached to an iPad (3rd generation iPad, Apple, Cupertino, CA) using the Audio Function Generator Pro application. The ambient noise decibel level was set to approximately 80 dBA as measured by the standard dosimeter. Testing was completed over multiple days so there was variability in the ambient noise level. Approximately 80 dBA were chosen because it was a level that could be found at a workplace that could interfere with communication, justifying the use of the radio, but would not require the use of hearing protection. To send pink noise through the radio, pink noise was emitted from an iPad and transmitted to the two-way radio hand-held microphone outside of the sound booth. Every 30 seconds, the hand-held radio microphone key was released and then depressed again to avoid the warning beep that discharged from the hand-held radio microphone if keyed for more than one minute. Each condition was measured for three minutes, and each condition was measured three times.
Prototype Correction Step Two
The correction was not designed to be applied to the time-weighted average (TWA) of the exposure but instead to only the individual one-minute measurements when the radio was transmitting. Step two of the correction identified which one-minute prototype measurements required the mathematical correction.
The raw prototype one-minute measurements are noticeably greater than the standard dosimeter one-minute measurements when noise is being transmitted through the radio in an environment with ambient noise levels less than 80 dBA. However, in work environments where ambient noise levels are 80 dBA and greater, or the environment is highly variable, identifying when the radio is used is not obvious because the dosimeters used in this study averaged decibel levels over one minute. In these more challenging workplaces, a difference value must be used to help identify when the difference is a real difference or just a result of the minute averaging of the dosimeters.
To determine the difference value, the prototypes were taken into an industrial setting with ambient noise close to 80 dBA. The work setting was chosen so that identifying the difference value would be a challenge of the prototypes, but wouldn’t require the workers to wear hearing protection, which the two-way radio earpiece was not verified to provide.
Both prototypes were brought into a mechanical sorting facility. The facility was a large building with the majority of the area dedicated to mechanical sorting using conveyors. Offices were located in a small central portion of the sorting floor as well as on one side of the building. The conveyors were only used during the sorting period of a shift, which lasted between three to five hours depending on the amount of material being sorted. Laborers and managers at the facility wore two-way radios during sorting to communicate due to the size of the facility and the ambient noise levels (greater than 80 dBA) produced by the conveyors. Laborers stayed in the sort area. Managers moved through the entire facility.
Two managers wore a prototype, one wore an attachment and one wore a microphone, and a standard dosimeter (Edge 5, 3M, St. Paul, MN, USA) for the first two hours of a sort while completing their normal activities. During the two hours, a third radio at 100% radio setting was put in a quiet room where there were no other noise sources above 80 dBA, with an additional Edge dosimeter (Edge 5, 3M, St. Paul, MN, USA). All dosimeters were programmed to American Conference of Governmental Industrial Hygienists (ACGIH) specifications of A weighting, 3 dB exchange rate, and 80 dB threshold (Berger, 2003). By enabling the 80 dBA threshold, the recorded one-minute measurements showed when noise was transmitted through the radio. Whenever there was not a transmission through the radio, the dosimeter in the room recorded a value of 0.00 dBA. When a transmission was sent through the radio, the noise in the room increased to over 80 dBA so a number greater than 0.00 was recorded.
The five dosimeters were started as close as possible to the same starting time. The microphone prototype and associated standard dosimeter were started 19 seconds after the room dosimeter. The attachment prototype and associated standard dosimeter were started 10 seconds after the room dosimeter. The manager who wore the attachment prototype needed to have the prototype adjusted so the prototype was paused for five minutes during the two hours. After the two hours were complete, the dosimeter and prototypes were downloaded as one-minute measurements and the data was analyzed.
Data Analysis
First, the number of one-minute measurements from the room dosimeter that were anything other than 0.00 dBA were summed to find the total number of minutes the radio was used. Then, the one-minute measurements for each workers’ standard dosimeter (Li_standard) and prototype (Li_prototype) were matched by the time stamp. The differences (Di) of each minute i were calculated using equation 3.
Di = Li_prototype – Li_standard [3]
The number of minutes where Di was greater than the tested difference value was summed and then divided by the total number of minutes the radio was used. Six values to be compared to Di as the difference values were: 8 dBA, 9 dBA, 10 dBA, 11 dBA, 12 dBA and 13 dBA. A percentage of the correctly identified minutes was compared for each difference value.
The total number of minutes the radio was used over the two-hours was 80 minutes. The minute volume levels measured when a transmission was sent through the radio varied from 59.9 dBA to 94.2 dBA. Every minute value of the dosimeter was averaged over the 60 seconds of the minute. Therefore, if a comment through the radio did not last for a full minute, the decibel value for the minute recorded by the dosimeter was less than the threshold value of 80 dBA. The range of decibel levels showed that some radio transmission noise lasted for less than a minute while others lasted for the full minute.
The test values to compare to Di are shown in Table 1. The test value of 10 dBA had the largest percentage without the percentage exceeding 100%, so it was chosen as the difference value.
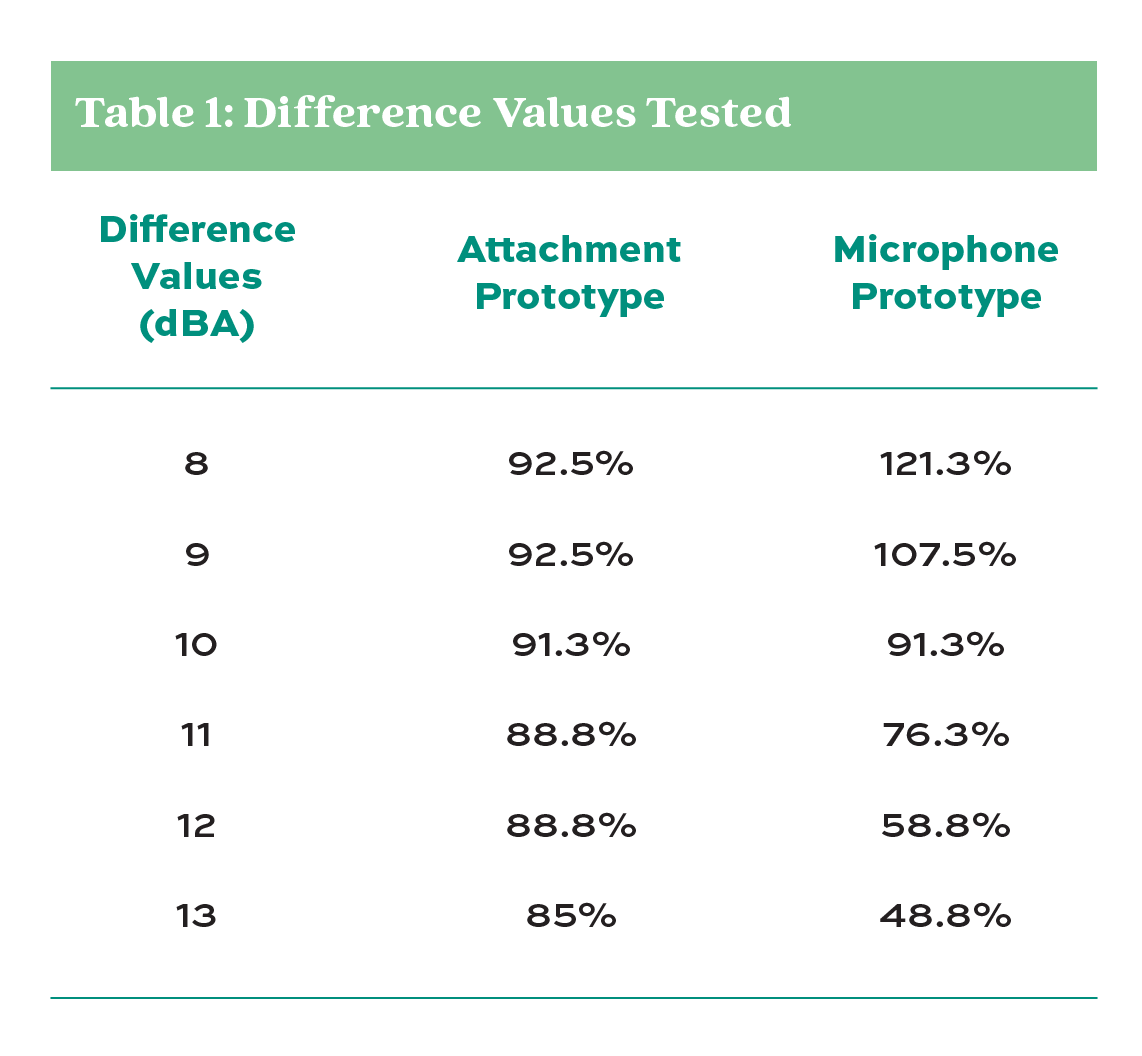
Workplace Trials
Both prototypes were utilized at two workplaces. The first workplace was the mechanical sorting facility where step two of the correction was completed. Radios were only worn while sorting occurred, so exposures were only measured during the sorting period. Hearing protection was not provided by the facility for any of the workers. Participants included four laborers and four managers (one female and seven males) ages 20-59 years (mean 31.7 years). One laborer and one manager dropped out during the course of the field trial. When using a two-way radio during a sorting shift, the workers either wore a radio with a prototype or the worker wore a radio with a shoulder-mounted radio speaker. Three shifts were measured for each worker and a different radio configuration was randomly assigned to each shift (standard dosimeter and attachment prototype, standard dosimeter and microphone prototype, or standard dosimeter with a second standard dosimeter on the same shoulder as a shoulder-mounted radio speaker).
The second workplace was a university campus where police officers wore the prototypes during a training traffic stop scenario. The ambient noise during the scenario was less than 80 dBA. The scenario was conducted where a large parking lot and campus streets intersected, and civilian traffic was restricted. Four officers (one female and three males) were involved in the scenario. One officer served as the contact officer. A second served as the cover officer. A third officer occupied and operated the suspect vehicle. The fourth officer served as the dispatcher. The scenario started with the contact and cover officers in the marked squad conducting stationary radar in the campus parking lot. The officers visually identified a speeding suspect vehicle and activated the wail siren. It took approximately one block to stop the suspect vehicle. The contact officer approached the driver side while the cover officer remained at the rear passenger corner of the suspect vehicle. The scenario included routine practices for interacting with dispatch, officers, and suspects. The contact and cover officers conducted the scenario three times with each prototype.
For both workplaces, all the dosimeters and prototypes were programmed to ACGIH specifications. Before any measurements were collected, each participant completed a hearing screen (Earscan 3 Manual Audiometer ES3M, Micro Audiometrics Corporation, Murphy, NC). Sound levels in testing locations met the American National Standard Criteria for maximum permissible ambient noise levels for audiometric test rooms (ANSI, 1999). Three of the participants did not pass the hearing screen in one ear. All participants self-reported normal hearing. All participants were instructed to select the radio setting normally used during a shift. After participants wore the prototypes, they were given a questionnaire asking about comfort and fit.
Results
Correction Step One Laboratory Testing
Table 2 shows the results of the correction step one laboratory testing. When compared to the dosimeter equivalent, the corrected microphone prototype was more accurate than the corrected attachment prototype with a mean difference of -0.2 dBA and -6.9 dBA, respectively. However, both corrected prototypes were consistent across tests with the microphone prototype standard deviation of 0.76 dBA and the attachment prototype standard deviation of 1.26.
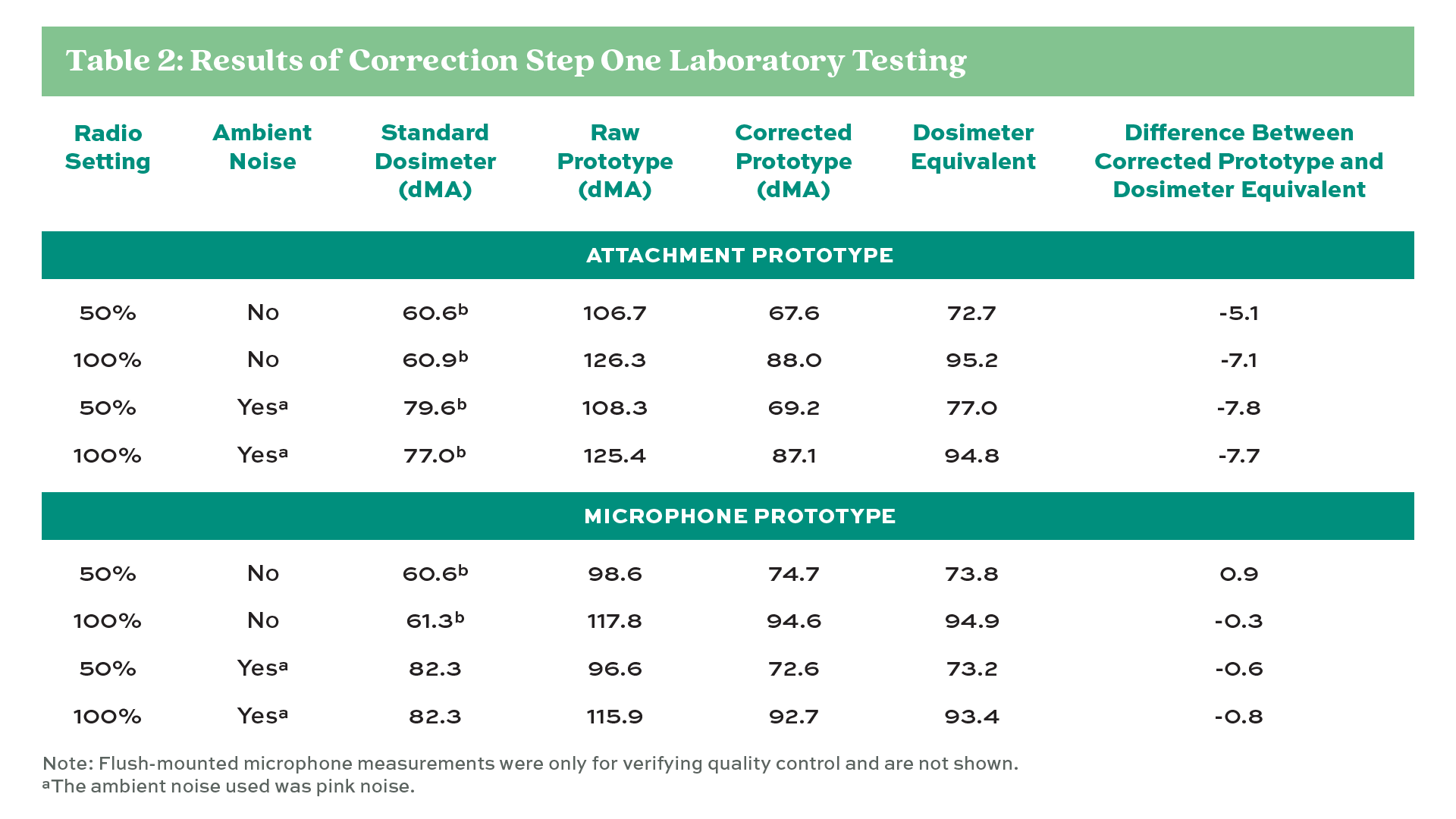
Workplace Trials
Sorting Facility
The descriptive data from the sorting facility are shown in Table 3. Statistical significance was not calculated due to the small sample size. The mean standard dosimeter measured noise exposure was similar for both managers (85.7-87.0 dBA) and laborers (85.7-87.4 dBA). The corrected prototypes measured differences less than 2 dBA from the standard dosimeter. The corrected prototype levels were 3.6-7.9 dBA less than the shoulder speaker.
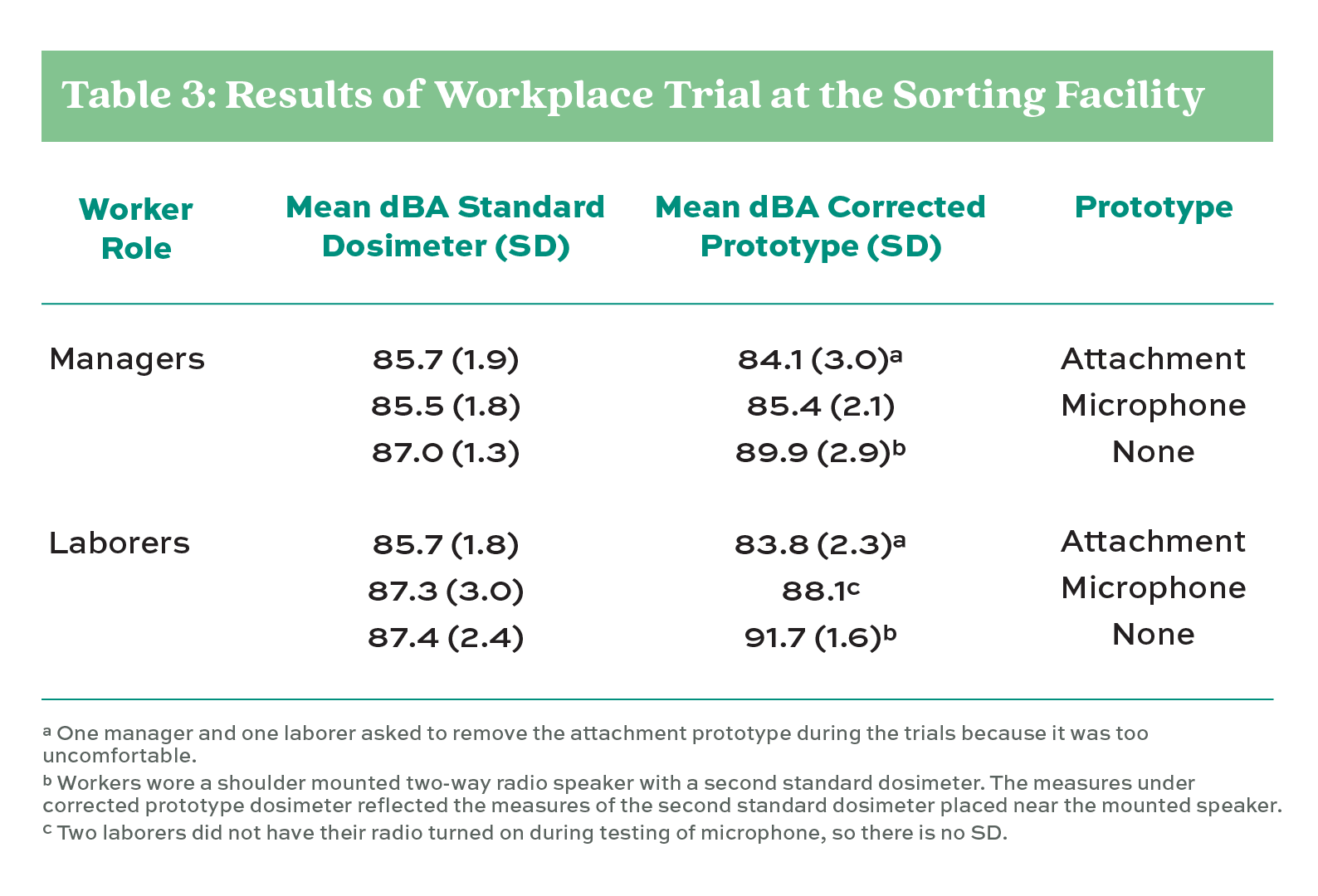
When surveyed, one participant reported tinnitus after the shift. All participants self-reported 100% radio setting for the majority of their shift and a preference for the microphone prototype. Participants that wore the attachment prototype indicated that the earpiece required readjusting two to three times during the shift. One laborer and one manager requested to remove the attachment prototype because it was uncomfortable and interfered with work.
Traffic Stop Scenario
The descriptive data from the traffic stop scenario are shown in Table 4. Statistical significance was not calculated due to the small sample size of n=3. Both officers adjusted their radio setting during the scenarios. The contact officer preferred a radio setting around 50%, while the cover officer preferred a radio setting near 100%. The contact officer experienced higher mean standard dosimeter levels than the cover officer. Both prototypes identified that the cover officer measured corrected prototype mean noise levels of 8 or 12 dBA above the standard dosimeter. Both officers preferred the microphone prototype over the attachment prototype for comfort and usability.
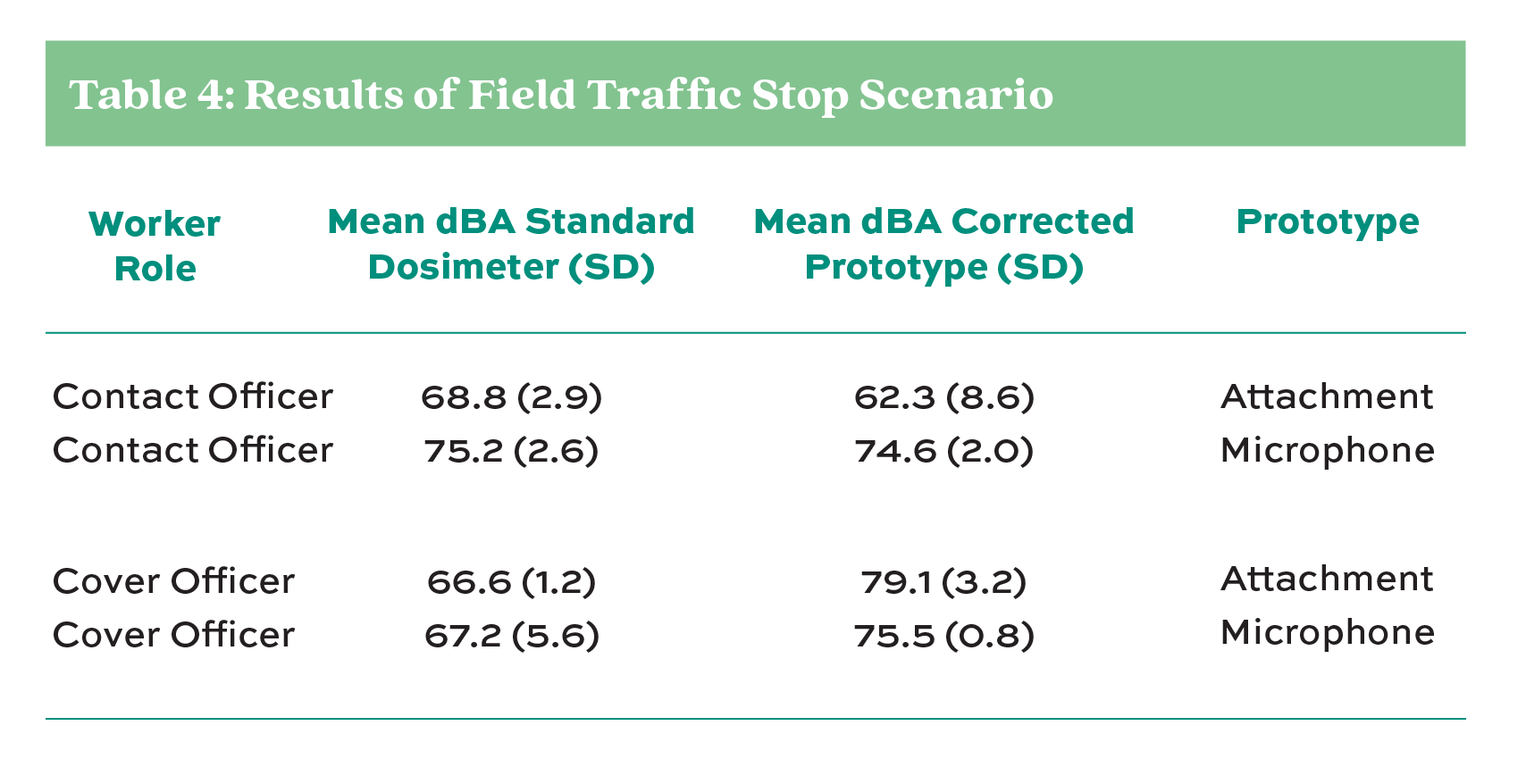
Discussion
The previously documented and standardized methods for measuring noise exposure in occupational settings with two-way radio communication are limited, and consultants with the equipment capabilities to follow the standard recommendations are sparse. This pilot work indicated that the prototypes may fill the noise measurement need and, with refinement, may serve as an affordable option for measuring two-way radio noise exposure from an earpiece in unpredictable and physically demanding work environments.
Which prototype an individual would select would depend on needs and resources. The microphone prototype was the most accurate and comfortable. Unless safety professionals are comfortable with electrical and mechanical engineering tasks, this prototype serves more as a model for fabrication by a noise dosimeter manufacturer as a potential adaptation to an existing product. The attachment prototype was not as accurate, but it was less expensive and most intuitive to fabricate. It required access to a 3-D printer and purchase of extra tubing and adhesive. Given that the attachment was not as accurate, individuals who use this prototype would want to consider the 6.9 dBA difference from corrected levels when interpreting results. Even so, the attachment would be an inexpensive option for exploring a noise issue before hiring a consultant.
In addition to illustrating the prototype potential for measuring noise in an earpiece caused by a two-way radio, the data presented highlights the importance of measuring three interacting variables when considering noise exposure from two-way radios: ambient noise, radio setting preference, and percentage of radio communication for a given occupational task. Table 3 shows that at the sorting facility with higher levels of ambient noise, the radio earpiece appeared to be protective in the ear that it is worn. High levels of ambient noise combined with high levels of radio use with a shoulder-mounted radio speaker led to the highest risk of increased noise exposure. The data from the traffic stop scenario (Table 4) emphasizes the influence of personal radio setting preference and occupational tasks. The contact officer, with a lower radio setting preference, experienced higher levels of ambient noise positioned closer to the suspect vehicle and relied more heavily on verbal communication with the suspect. The cover officer, with a higher radio setting preference, was further from the vehicle noise and relied more heavily on radio communication with the contact officer and dispatch. These noted differences in listening preferences and occupational tasks may explain variations in hearing health.
It is important to recognize that this pilot study had a small sample size and limited testing conditions. The current project evaluated a single source of ambient and radio noise in the laboratory. In the field, only two occupational settings were evaluated with a small set of workers. The prototypes, like the standard noise dosimeter used in this study, do not collect ⅓ octave band spectral information, making it difficult to calculate the dosimeter equivalents without assuming the laboratory data mirrors the field data. Additionally, the dosimeters only capture data in one-minute intervals. Radio communication does not necessarily take the entire minute. In order to generalize the results of this pilot study, future work should include a more robust sample size and investigate a wider range of test conditions. A collaborative partnership with an existing dosimeter manufacturer would likely improve the prototype design and result in a more affordable market-ready solution.
Conclusion
The prototypes were able to explore the impact of two-way radio communication on noise exposure in unpredictable and physically demanding work environments. The microphone prototype was more accurate and comfortable, while the attachment prototype was simpler and affordable to create. When determining the impact two-way radio communication has on hearing health, the results encourage measuring the intersection of radio setting preference, ambient noise, and amount of time a radio is used during an occupational task.
References
American National Standards Institute (ANSI), (1999). American national standard criteria for permissible ambient noise during audiometric testing. American Institute of Physics.
Berger, E.H. ed., (2003). The noise manual. AIHA.
Brammer, A. J., Yu, G., Bernstein, E. R., Peterson, D. R., Cherniack, M. G. & Tufts, J. B. (2009). Monitoring sound pressure at the eardrum for hearing conservation. Canadian Acoustics, 37(3), 108-109.
Dajani, H., Kunov, H. & Seshagiri, B. (1996). Real-time method for the measurement of noise exposure from communication headsets. Applied Acoustics, 49(3), 209-224.
Giguère, C., Behar, A., Dajani, H. R., Kelsall, T., & Keith, S. E. (2012). Direct and indirect methods for the measurement of occupational sound exposure from communication headsets. Noise Control Engineering Journal, 60(6), 630-644.
Gilbertson, L.R. and Vosburgh, D. J. H. (2015). Patrol officer daily noise exposure. Journal of Occupational and Environmental Hygiene, 12(10), 686-691.
Gilbertson, L., Vosburgh, D. J. H., and Klein, T. (2020). U.S. Patent No. 10,560,776. Washington, DC: U.S. Patent and Trademark Office. Available from: http://patft.uspto.gov/netacgi/nph-Parser?Sect1=PTO1&Sect2=HITOFF&d=PALL&p=1&u=%2Fnetahtml%2FPTO%2Fsrchnum.htm&r=1&f=G&l=50&s1=10,560,776.PN.&OS=PN/10,560,776&RS=PN/10,560,776
Ide, C.W. (2011). Hearing losses in wholetime firefighters occurring early in their careers. Occupational Medicine, 61(7), 509-511.
ISO, (2002). Acoustics- determination of sound immission from sound sources placed close to the ear-Part 1: Technique using a microphone in a real ear MIRE technique (11904-1) [Standard]. International Organization for Standardization.
ISO, (2004). Acoustics-determination of sound immission from sound sources placed close to the ear—Part 2: Technique using a manikin (11904-2) [Standard]. International Organization for Standardization.
Keith, S. E., Michaud, D. S. and Chiu, V. (2008). Evaluating the maximum playback sound levels from portable digital audio players. The Journal of the Acoustical Society of America, 123(6), 4227-4237.
Killion, M. C. (2002). New thinking on hearing in noise: A generalized articulation index. Seminars in Hearing, 23(1), 057-076.
Kunov, H., Giguere, C. & Simpson, R. (1989). Method for measuring noise exposure from communications headsets. Proceedings of the Annual Meeting of the Canadian Acoustical Association, 16-19.
Lesage, F.X., Jovenin, N., Deschamps, F. & Vincent, S. (2009). Noise-induced hearing loss in French police officers. Occupational Medicine, 59(7), 483-486.
Muchnik, C., Amir, N., Shabtai, E. & Kaplan-Neeman, R. (2012). Preferred listening levels of personal listening devices in young teenagers: Self reports and physical measurements. International Journal of Audiology, 51(4), 287-293.
Nélisse, H., Le Cocq, C., Boutin, J., Laville, F. & Voix, J. (2015). Systematic evaluation of the relationship between physical and psychoacoustical measurements of hearing protectors’ attenuation. Journal of Occupational and Environmental Hygiene, 12(12), 829-844.
OSHA technical manual, (2013). Evaluating Noise Exposure of Workers Wearing Sound-generating headsets. U.S. Dept. of Labor, Occupational Safety and Health Administration [Internet] [cited 2020 Jul 17]. Available from: https://www.osha.gov/otm/section-3-health-hazards/chapter-5
Portnuff, C. D., Fligor, B. J. and Arehart, K. H. (2013). New measurement techniques for portable listening devices: Technical Report. Journal of the Audio Engineering Society, 61(10), 749-754.
Shotland, L. I. (1996). Dosimetry measurements using a probe tube microphone in the ear canal. The Journal of the Acoustical Society of America, 99(2), 979-984.
Tubbs, R. L. (1995). Noise and hearing loss in firefighting. Occupational Medicine, 10(4), 843.
Taylor, B. & Teter, D. (2009). Ear molds: Practical considerations to improve performance in hearing aids. Hearing Review, 16(10), 10-14.